Exoplanet Discovery Calculator
The Nobel Prize in physics 2019 was apportioned to Michel Mayor and Didier Queloz's exoplanet discovery. As a tribute to their achievement and to help you understand how they did it, we present to you the Exoplanet Discovery Calculator, a tool that uses the same calculations researchers use to discover new exoplanets. We have baked into this tool 3 different detection methods (including the one that has just received a Nobel Prize) as well as some of the most interesting known pairs of star-exoplanet. With this calculator you will finally understand how new exoplanets are discovered, why has it been so hard to find them historically, and why it deserved this year's Nobel Prize in Physics. And as a bonus, we will show you how to use it to discover new (real or otherwise) planets. Let's jump right in!
Have you ever wondered, can aliens see you? 👁👁 Do they know about the Earth and the humans? Discover how with Álvaro in our video!
What is an exoplanet?
Are we alone in the universe? Since we can't just send a text message to "the universe", we have to come up with more ingenious ways to answer this question. The first important step is to ask ourselves: What are the conditions that allow life to exist? Obviously, one of the first conditions is that it needs a safe place to exist in. This safe place takes the form of a planet (more precisely, a habitable exoplanet, as we will see later).
Immediately we find ourselves pointing our telescopes to our neighbors in the Solar System. But that gives us at most 8 options, of which almost all get immediately excluded due to their characteristics. So, before we travel across space, we might want to answer the question: Are there any planets exogenous to our solar system? That is, do (habitable) exoplanets exist?
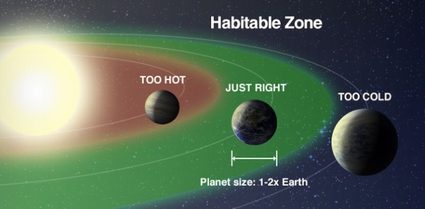
As you have probably figured out by now, the answer is yes (otherwise, this calculator wouldn't exist). In fact, we have now narrowed down the requirements for an exoplanet to be considered a "habitable exoplanet" based on the conditions necessary for life to exist. The requirements are expressed in terms of the orbiting distance from the main star, the presence of an atmosphere, and the composition of the planet that wouldn't instantly kill any form of life that we currently know.
When we apply these filters, we get a significant number of planets (at least "big" in terms of our current observational capabilities) that fall into the "habitable exoplanets" category. However, finding them proved to be much more tricky than just pointing our telescopes to the stars and look thoroughly.
A bit of history and trivia
The biggest paradigm shift ever in astronomy was the discovery of other galaxies outside of the Milky Way. The discovery is less than 100 years old, which is very recent if we consider how long we've been looking at the stars. It showed us that there is nothing special about our planet, Sun, or galaxy. The inevitable conclusion is that if our Sun is just like any other star, we should expect to *find planets orbiting most (if not all) other stars.
And that's precisely what scientists did, only using fancier techniques than using just our eyes. The results first appear in 1988 when the first exoplanet was found, though not even the researchers believed they had found a planet. This particular discovery was not confirmed until 2002, and at the time, it went by as possibly another small star.
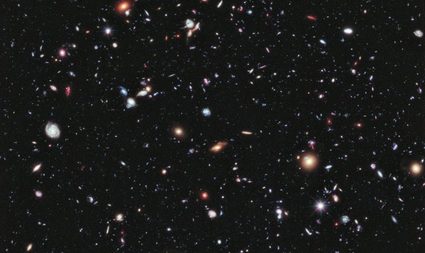
Mayor's and Queloz's discovery, in 1995, was the first confirmed exoplanet discovery and kick-started what could be described as a race to find as many exoplanets as possible. To date, there are more than 4100 exoplanets discovered. Their names and characteristics can be found in any of the
available online. For the trivia fans among you, here is a short exoplanets list with some of the more interesting ones (including some habitable exoplanets for good measure):-
TrES-4 — the largest exoplanet discovered so far.
-
Kepler-1520 b — a disintegrating planet?
-
HR 2562 b — the heaviest exoplanet.
-
Gliese 436 b — the first "hot-Neptune" discovered.
-
Phobetor — a "super-earth" or "hot-Neptune".
-
Dimidium (51 Pegasi b) — got their discoverers a Nobel Prize.
-
Proxima Centauri b — closest exoplanet known, potentially habitable exoplanet.
-
Dimidium (51 Pegasi b) — got their discoverers a Nobel Prize.
-
Tadmor (Gamma Cephei b) — first exoplanet to be discovered (not confirmed until 2002).
A fun fact about the names of exoplanets is that they always follow the same naming convention. The first string of characters (that might seem random to many) denotes the star system to which it belongs. That text is followed by a letter a
for the star around which the planets orbit (which is often omitted), and the rest of the letters b, c, d...
denote the order of discovery of the planets.
Nobel prize in physics 2019
Just a few days before writing this text, the Nobel Prize in Physics (2019) was awarded to 3 physicists “for contributions to our understanding of the evolution of the universe and Earth’s place in the cosmos”. Half of this prize was awarded to James Peebles (from Princeton University, USA) for “theoretical discoveries in physical cosmology” and the other half jointly awarded to Michel Mayor and Didier Queloz (from the University of Geneva, Switzerland) for “the discovery of an exoplanet orbiting a solar-type star”.
This discovery occurred in October 1995, when both researchers discovered Dimidium, also known as 51 Pegasi b, a planet orbiting the star 51 Pegasi (because following nomenclature rules strictly is something you aspire to do, not something you always do). It was found using the radial velocity technique, which is one of the most powerful methods we have nowadays. We will explain what it entails in more detail in the next section.
This might seem to some like too small of an achievement to be awarded the highest recognition in physics, but rest assured, this is a worthy milestone. Nobel Prizes are always a bit controversial, mainly because they have to be awarded for single achievements officially. They become less controversial when one considers these individual achievements as a representative event of a big revolution in a field.
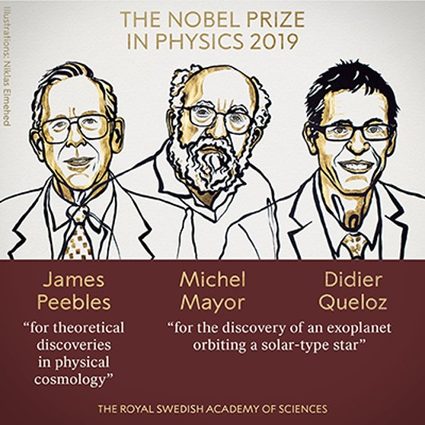
For example, even if we would admit that there's some luck involved in finding an exoplanet (much less than you think, though), it is undeniable that obtaining scientific evidence that there are other planets out there that can host life is an important breakthrough. If we add to this that, in real life, discoveries like this are not by chance but require decades of hard work and vast amounts of knowledge on very complex topics, it is clear that this Nobel Prize is properly justified.
And to pay tribute to these researchers and their achievements, we will look closer into what it takes to discover an exoplanet, in a very interactive way. For starters, let's take a look at some of the most successful methods of finding exoplanets.
The radial velocity method
We should start with the method that got the Nobel Prize: the radial velocity method. It is also called the redshift method or Doppler shift method for reasons that will become apparent in a moment. The first thing you need to know is what is redshift or the Doppler effect.
We also have our separated redshift calculator for you to better understand this.
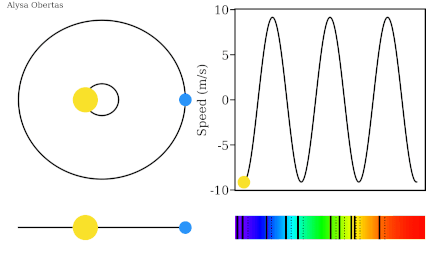
Very generally, this method refers to the change in wavelength that a wave experiences when it is emitted from a source that is moving with respect to the observer. It is most easily understood as the change in sound when a police siren approaches and then passes you - the difference in the pitch is due to Doppler effect.
When we are talking about the Doppler effect regarding light, we can call it redshift or blueshift. Redshift refers to the object moving away, making the wavelength longer (more "to the red" if we're talking about visible light), while blueshift refers to the object getting closer (more "to the blue"). There are other reasons why light might get red/blue-shifted, like the expansion of the universe or the presence of a strong gravitational field, but those are topics for another time.
When we are talking about the Doppler effect in relation to the velocity of the object, we can approximately define the amount of variation between the original color and the perceived color by using the following formula:
z = v/c
Where v
is the speed of the object with respect to us, c
is the speed of light (which is much greater than v
), and z
is called redshift, and it's a dimensionless quantity a.k.a. it doesn't have any units. To calculate the redshifted wavelength, called λ_reds
, we simply apply the formula:
λ_reds = (1 + z) × λ_og
where λ_og
is the original wavelength emitted by the star.
If all this sounds complicated, don't worry. For our exoplanet detection, all you need to know is that this effect is related to the speed of the object with respect to the observer, and by measuring it, we can calculate the speed of an object far away, like a star very very very far away.
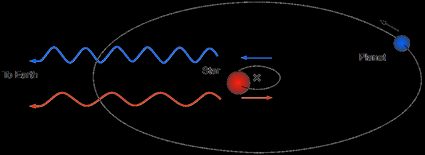
It is not commonly mentioned in high-school physics, but not only do planets orbit stars, but stars do also have an orbit 'around' the planet. It is generally so small that the center of the orbit lies inside the star itself, and, for most purposes, it is negligible. It's not for us. See our orbital period calculator to learn more!
This movement of the star can be observed directly (see the Astrometry method section for more information). Still, it can also be measured by observing how much the red/blue-shift of the light of the star changes. This effect usually is very tiny; however, it has been one of the most historically successful methods, with almost 780 discoveries already!
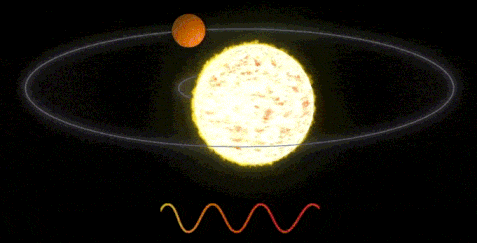
The radial velocity method is especially good at detecting massive planets around small stars since these situations produce the highest radial speeds of a star and, hence, the biggest Doppler shift.
The transit method
Next up is the transit method, by far the easiest to understand since it could also be called the "eclipse" method and remain an accurate description of the process.
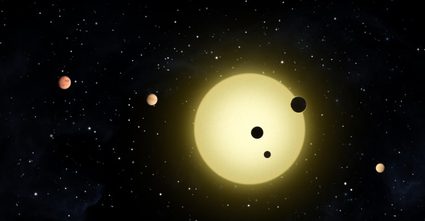
This method relies heavily on the plane of the orbit of the planet being aligned with the line of vision we have from the Earth, but if this happens, wonderful things can be seen. This is by far the most successful method, and has already discovered more than 3100 planets so far. And the best part is that it is based on the same principle that helps you block the light of the sun with your hand on an extremely sunny day: solid objects block light.
Provided the orbit of the planet is aligned with our line of vision, once per orbit, the planet will be between the star and us creating an eclipse of sorts. The star is so far away that we cannot see it like we can see lunar and solar eclipses. Still, with the appropriate instruments (very powerful telescopes), we can detect the transit of the planet in front of the star as a slight decrease in the star's brightness. This dip in brightness depends on the relative size of the planet and the star.
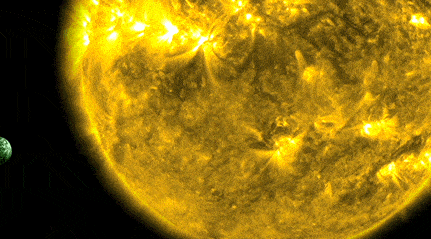
This method is very good at finding very big planets of almost any orbit radius as long as the plane of translation contains the line of vision from the Earth to the star.
Astrometry method
Another method that can be used to detect exoplanets is the astrometry method, which looks for different effects of the same phenomenon as the radial velocity method. In this case, instead of looking for indirect evidence of a star wiggling, scientists look for the actual movement of a star.
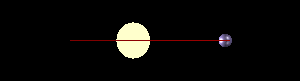
It doesn't need to be said how hard it is to actually measure these small changes in the position of a star that is so far away that we can't really see it. No wonder this technique has
.By using the calculator, you can try to understand why it is so hard as the angular units used to measure this wobble are narcsec (nanoseconds of arc) - a millionth of which are an arcsec, which in turn is 3600th of a degree. For reference, a finger occupies about one degree of angle in your vision if you extend your arm as far as you can; a narcsec is 3.6 billionth of that!
See more in our angle conversion calculator.
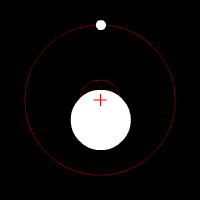
The other methods (gravitational microlensing and direct imaging)
There are many other methods that can be used to discover exoplanets, in fact, 2 more have already been successfully used: gravitational microlensing and direct imaging. The later is exactly what it sounds like, point your telescope at a star-system and see if there's any planet orbiting it.
There are obviously many more steps involved in these observations, like researching which stars are more likely to have planets around them, selecting the ones that are close enough to be observed, and then the actual data-taking process. We tend to overlook the complexity of this last step, thinking that is just a simple observation/picture and we're done. In reality, "seeing" a planet involves days of waiting for the right weather conditions, painstakingly taking data, then analyzing it with very complex algorithms until you finally get something usable.
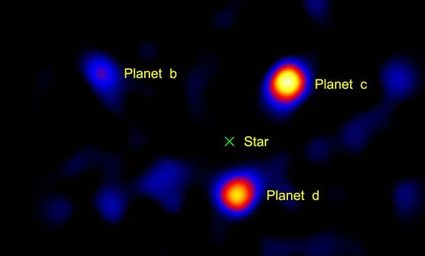
You can probably guess that direct imaging is not the most effective method for finding new exoplanets. So far, we have found 47 using this method. There is another method that has yielded almost double this number: gravitational microlensing.
According to the theory of general relativity, any mass distorts space-time in a way that light passing by such objects gets slightly bent. This effect is most noticeable around very small and very massive objects, like black holes and, to a lesser extent, stars.
However, these effects also occur when planets are involved, so, with very precise instruments and very clever processing algorithms, we can see and detect these slight differences in light paths to discover new planets orbiting stars. We have found 83 to date, and we will likely continue to discover more as we improve and then refine the technique.
How to use the exoplanet discovery Omni-calculator?
All this might have seemed dense and complicated (if you did not jump directly here), so it's time to get hands-on and explore each of the techniques with our calculator. We have also included representations of the effects you could expect to see in real life to help you understand all of these methods of exoplanets discovery.
The first thing you should do is select the type of detection method you want to explore. Once you've selected your favorite, you will be presented with the relevant data for said measurement. Among the fields you will find are:
-
Mstar — Mass of the star around which the planet orbits;
-
Rstar — Radius of the star around which the planet orbits;
-
Mplanet — Mass of the planet you wish to detect;
-
Rplanet — Radius of the planet you wish to detect;
-
Orbital Radius — Semi-major axis of the elliptical orbit of the planet around the star; and
-
Distance — Distance from the star to the Earth.
Each of these fields will appear/disappear as they become relevant/irrelevant for each type of measurement, and the results will be presented below in written form with any accompanying graphics. To make things easier, we have included a star and an exoplanet list with some of our favorite exoplanets and their corresponding stars (look for matching names). Feel free to use those presets, mix them up, or find your own values.
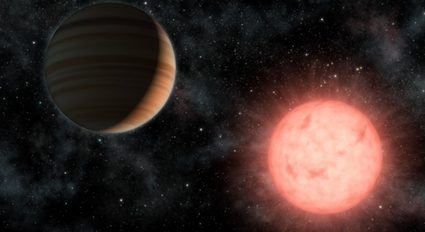
Pro-tip:
If you are looking for the parameters of stars and exoplanets, go for specialized online planet/star catalogs. You can find literally every single parameter ever measured for each exoplanet and their corresponding stars.
There is also an advanced-mode
that allows you to change and see some additional parameters of the star-planet system. Most importantly, you will find the option to be presented with the real data or with data modified to help you visualize the physical effects that help detect these exoplanets. Just remember, if you're gonna run out and buy a new telescope, make sure to get one similar to this:
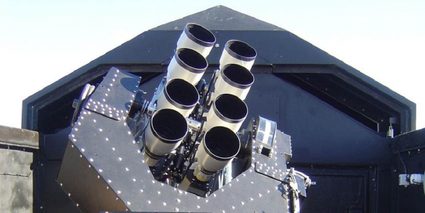
A (necessary) comment on the results
If you have already played around with the calculator, you might have noticed that some (if not all) of the results seem to be comically small, you might not even know how to put them into context. We're here to help.
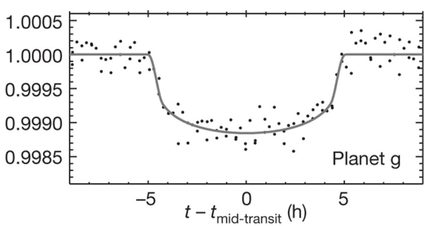
For both the Radial Velocity and Transient methods, you can check on your own what the percentage changes represent in real life by simply selecting Improve data visualization -> No
in the advanced mode and playing around with the values. For the Wiggle method, it's a bit more complicated but as a reference Alpha Centauri (the closest and third brightest star in the night sky) has an angular size of approximately 8.47 marcsec
(mili-arcsec). A nano-arcsec
is one-millionth of a marcsec
.
This brings us to the common feature of all the results: they are very small. So small indeed that you could not detect them with your naked eye (or through Earth's atmosphere). So that's why we have included the visual enhancing feature (CSI style, but for realsies) that allows you to get an idea of what the data would look like if you were sensitive enough to those super small changes.
Nevertheless, here at Omni, we are all about empowering people with real data and scientifically accurate knowledge, so we still give the option of turning it off completely so that you can see what the real value is. If you chose not to see the real values, then you can still get a grasp for the real situation because we include the factor by which the effects are amplified, which dynamically changes so that it doesn't depart too much from reality.
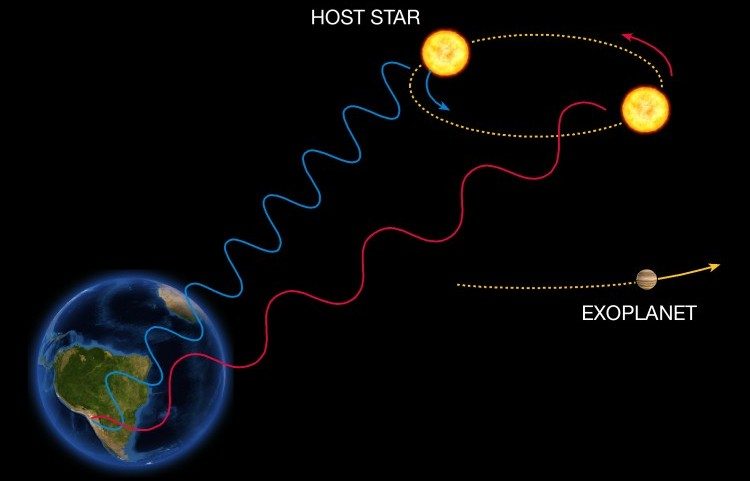
RedShifted light
BlueShifted light